mirror of
https://git.proxmox.com/git/mirror_zfs.git
synced 2025-06-30 21:17:35 +03:00
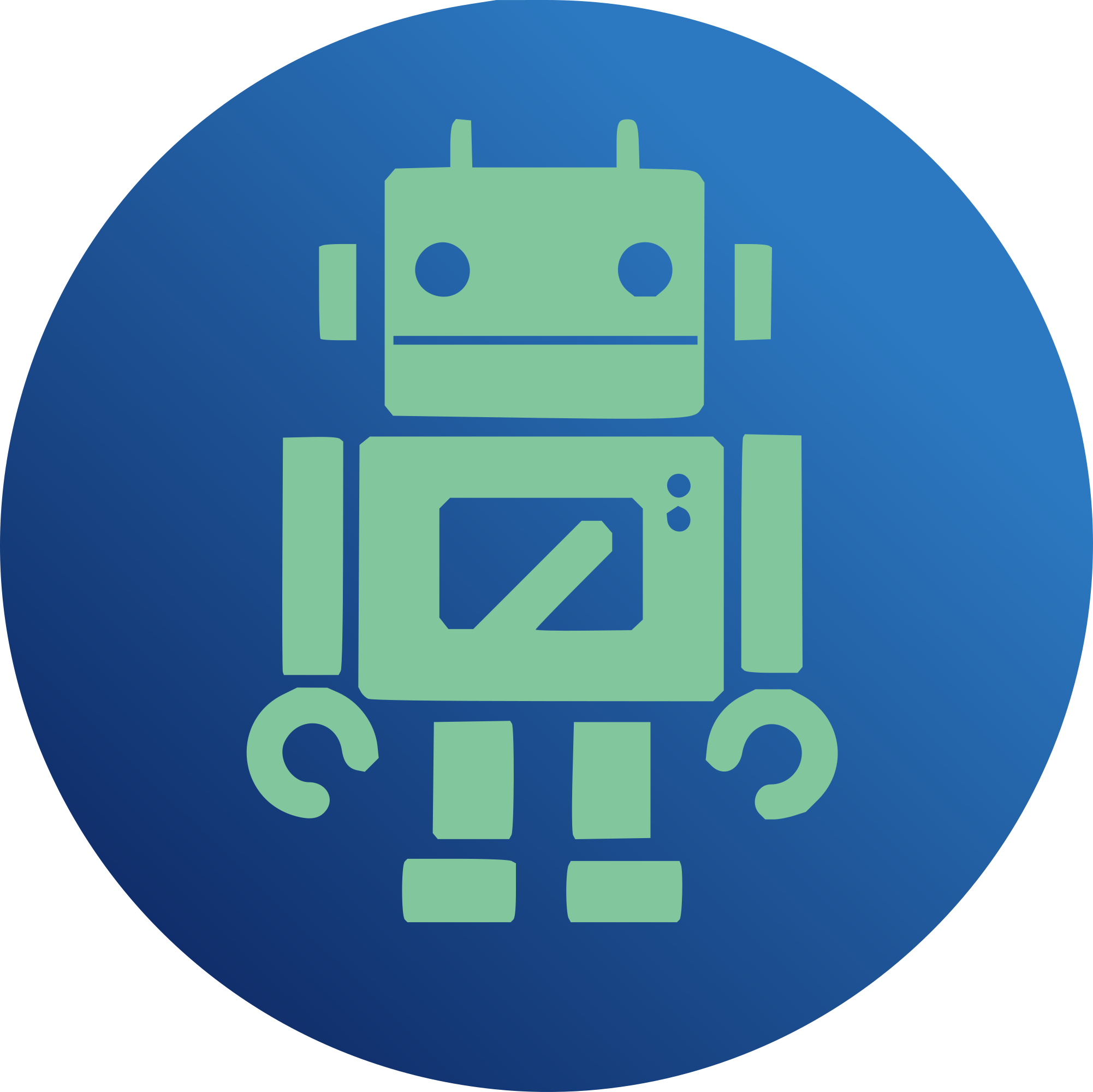
This patch implements a new tree structure for ZFS, and uses it to store range trees more efficiently. The new structure is approximately a B-tree, though there are some small differences from the usual characterizations. The tree has core nodes and leaf nodes; each contain data elements, which the elements in the core nodes acting as separators between its children. The difference between core and leaf nodes is that the core nodes have an array of children, while leaf nodes don't. Every node in the tree may be only partially full; in most cases, they are all at least 50% full (in terms of element count) except for the root node, which can be less full. Underfull nodes will steal from their neighbors or merge to remain full enough, while overfull nodes will split in two. The data elements are contained in tree-controlled buffers; they are copied into these on insertion, and overwritten on deletion. This means that the elements are not independently allocated, which reduces overhead, but also means they can't be shared between trees (and also that pointers to them are only valid until a side-effectful tree operation occurs). The overhead varies based on how dense the tree is, but is usually on the order of about 50% of the element size; the per-node overheads are very small, and so don't make a significant difference. The trees can accept arbitrary records; they accept a size and a comparator to allow them to be used for a variety of purposes. The new trees replace the AVL trees used in the range trees today. Currently, the range_seg_t structure contains three 8 byte integers of payload and two 24 byte avl_tree_node_ts to handle its storage in both an offset-sorted tree and a size-sorted tree (total size: 64 bytes). In the new model, the range seg structures are usually two 4 byte integers, but a separate one needs to exist for the size-sorted and offset-sorted tree. Between the raw size, the 50% overhead, and the double storage, the new btrees are expected to use 8*1.5*2 = 24 bytes per record, or 33.3% as much memory as the AVL trees (this is for the purposes of storing metaslab range trees; for other purposes, like scrubs, they use ~50% as much memory). We reduced the size of the payload in the range segments by teaching range trees about starting offsets and shifts; since metaslabs have a fixed starting offset, and they all operate in terms of disk sectors, we can store the ranges using 4-byte integers as long as the size of the metaslab divided by the sector size is less than 2^32. For 512-byte sectors, this is a 2^41 (or 2TB) metaslab, which with the default settings corresponds to a 256PB disk. 4k sector disks can handle metaslabs up to 2^46 bytes, or 2^63 byte disks. Since we do not anticipate disks of this size in the near future, there should be almost no cases where metaslabs need 64-byte integers to store their ranges. We do still have the capability to store 64-byte integer ranges to account for cases where we are storing per-vdev (or per-dnode) trees, which could reasonably go above the limits discussed. We also do not store fill information in the compact version of the node, since it is only used for sorted scrub. We also optimized the metaslab loading process in various other ways to offset some inefficiencies in the btree model. While individual operations (find, insert, remove_from) are faster for the btree than they are for the avl tree, remove usually requires a find operation, while in the AVL tree model the element itself suffices. Some clever changes actually caused an overall speedup in metaslab loading; we use approximately 40% less cpu to load metaslabs in our tests on Illumos. Another memory and performance optimization was achieved by changing what is stored in the size-sorted trees. When a disk is heavily fragmented, the df algorithm used by default in ZFS will almost always find a number of small regions in its initial cursor-based search; it will usually only fall back to the size-sorted tree to find larger regions. If we increase the size of the cursor-based search slightly, and don't store segments that are smaller than a tunable size floor in the size-sorted tree, we can further cut memory usage down to below 20% of what the AVL trees store. This also results in further reductions in CPU time spent loading metaslabs. The 16KiB size floor was chosen because it results in substantial memory usage reduction while not usually resulting in situations where we can't find an appropriate chunk with the cursor and are forced to use an oversized chunk from the size-sorted tree. In addition, even if we do have to use an oversized chunk from the size-sorted tree, the chunk would be too small to use for ZIL allocations, so it isn't as big of a loss as it might otherwise be. And often, more small allocations will follow the initial one, and the cursor search will now find the remainder of the chunk we didn't use all of and use it for subsequent allocations. Practical testing has shown little or no change in fragmentation as a result of this change. If the size-sorted tree becomes empty while the offset sorted one still has entries, it will load all the entries from the offset sorted tree and disregard the size floor until it is unloaded again. This operation occurs rarely with the default setting, only on incredibly thoroughly fragmented pools. There are some other small changes to zdb to teach it to handle btrees, but nothing major. Reviewed-by: George Wilson <gwilson@delphix.com> Reviewed-by: Matt Ahrens <matt@delphix.com> Reviewed by: Sebastien Roy seb@delphix.com Reviewed-by: Igor Kozhukhov <igor@dilos.org> Reviewed-by: Brian Behlendorf <behlendorf1@llnl.gov> Signed-off-by: Paul Dagnelie <pcd@delphix.com> Closes #9181
1031 lines
32 KiB
C
1031 lines
32 KiB
C
/*
|
|
* CDDL HEADER START
|
|
*
|
|
* The contents of this file are subject to the terms of the
|
|
* Common Development and Distribution License (the "License").
|
|
* You may not use this file except in compliance with the License.
|
|
*
|
|
* You can obtain a copy of the license at usr/src/OPENSOLARIS.LICENSE
|
|
* or http://www.opensolaris.org/os/licensing.
|
|
* See the License for the specific language governing permissions
|
|
* and limitations under the License.
|
|
*
|
|
* When distributing Covered Code, include this CDDL HEADER in each
|
|
* file and include the License file at usr/src/OPENSOLARIS.LICENSE.
|
|
* If applicable, add the following below this CDDL HEADER, with the
|
|
* fields enclosed by brackets "[]" replaced with your own identifying
|
|
* information: Portions Copyright [yyyy] [name of copyright owner]
|
|
*
|
|
* CDDL HEADER END
|
|
*/
|
|
/*
|
|
* Copyright 2009 Sun Microsystems, Inc. All rights reserved.
|
|
* Use is subject to license terms.
|
|
*/
|
|
|
|
/*
|
|
* Copyright (c) 2012, 2018 by Delphix. All rights reserved.
|
|
*/
|
|
|
|
#include <sys/zfs_context.h>
|
|
#include <sys/vdev_impl.h>
|
|
#include <sys/spa_impl.h>
|
|
#include <sys/zio.h>
|
|
#include <sys/avl.h>
|
|
#include <sys/dsl_pool.h>
|
|
#include <sys/metaslab_impl.h>
|
|
#include <sys/spa.h>
|
|
#include <sys/spa_impl.h>
|
|
#include <sys/kstat.h>
|
|
#include <sys/abd.h>
|
|
|
|
/*
|
|
* ZFS I/O Scheduler
|
|
* ---------------
|
|
*
|
|
* ZFS issues I/O operations to leaf vdevs to satisfy and complete zios. The
|
|
* I/O scheduler determines when and in what order those operations are
|
|
* issued. The I/O scheduler divides operations into five I/O classes
|
|
* prioritized in the following order: sync read, sync write, async read,
|
|
* async write, and scrub/resilver. Each queue defines the minimum and
|
|
* maximum number of concurrent operations that may be issued to the device.
|
|
* In addition, the device has an aggregate maximum. Note that the sum of the
|
|
* per-queue minimums must not exceed the aggregate maximum. If the
|
|
* sum of the per-queue maximums exceeds the aggregate maximum, then the
|
|
* number of active i/os may reach zfs_vdev_max_active, in which case no
|
|
* further i/os will be issued regardless of whether all per-queue
|
|
* minimums have been met.
|
|
*
|
|
* For many physical devices, throughput increases with the number of
|
|
* concurrent operations, but latency typically suffers. Further, physical
|
|
* devices typically have a limit at which more concurrent operations have no
|
|
* effect on throughput or can actually cause it to decrease.
|
|
*
|
|
* The scheduler selects the next operation to issue by first looking for an
|
|
* I/O class whose minimum has not been satisfied. Once all are satisfied and
|
|
* the aggregate maximum has not been hit, the scheduler looks for classes
|
|
* whose maximum has not been satisfied. Iteration through the I/O classes is
|
|
* done in the order specified above. No further operations are issued if the
|
|
* aggregate maximum number of concurrent operations has been hit or if there
|
|
* are no operations queued for an I/O class that has not hit its maximum.
|
|
* Every time an i/o is queued or an operation completes, the I/O scheduler
|
|
* looks for new operations to issue.
|
|
*
|
|
* All I/O classes have a fixed maximum number of outstanding operations
|
|
* except for the async write class. Asynchronous writes represent the data
|
|
* that is committed to stable storage during the syncing stage for
|
|
* transaction groups (see txg.c). Transaction groups enter the syncing state
|
|
* periodically so the number of queued async writes will quickly burst up and
|
|
* then bleed down to zero. Rather than servicing them as quickly as possible,
|
|
* the I/O scheduler changes the maximum number of active async write i/os
|
|
* according to the amount of dirty data in the pool (see dsl_pool.c). Since
|
|
* both throughput and latency typically increase with the number of
|
|
* concurrent operations issued to physical devices, reducing the burstiness
|
|
* in the number of concurrent operations also stabilizes the response time of
|
|
* operations from other -- and in particular synchronous -- queues. In broad
|
|
* strokes, the I/O scheduler will issue more concurrent operations from the
|
|
* async write queue as there's more dirty data in the pool.
|
|
*
|
|
* Async Writes
|
|
*
|
|
* The number of concurrent operations issued for the async write I/O class
|
|
* follows a piece-wise linear function defined by a few adjustable points.
|
|
*
|
|
* | o---------| <-- zfs_vdev_async_write_max_active
|
|
* ^ | /^ |
|
|
* | | / | |
|
|
* active | / | |
|
|
* I/O | / | |
|
|
* count | / | |
|
|
* | / | |
|
|
* |------------o | | <-- zfs_vdev_async_write_min_active
|
|
* 0|____________^______|_________|
|
|
* 0% | | 100% of zfs_dirty_data_max
|
|
* | |
|
|
* | `-- zfs_vdev_async_write_active_max_dirty_percent
|
|
* `--------- zfs_vdev_async_write_active_min_dirty_percent
|
|
*
|
|
* Until the amount of dirty data exceeds a minimum percentage of the dirty
|
|
* data allowed in the pool, the I/O scheduler will limit the number of
|
|
* concurrent operations to the minimum. As that threshold is crossed, the
|
|
* number of concurrent operations issued increases linearly to the maximum at
|
|
* the specified maximum percentage of the dirty data allowed in the pool.
|
|
*
|
|
* Ideally, the amount of dirty data on a busy pool will stay in the sloped
|
|
* part of the function between zfs_vdev_async_write_active_min_dirty_percent
|
|
* and zfs_vdev_async_write_active_max_dirty_percent. If it exceeds the
|
|
* maximum percentage, this indicates that the rate of incoming data is
|
|
* greater than the rate that the backend storage can handle. In this case, we
|
|
* must further throttle incoming writes (see dmu_tx_delay() for details).
|
|
*/
|
|
|
|
/*
|
|
* The maximum number of i/os active to each device. Ideally, this will be >=
|
|
* the sum of each queue's max_active. It must be at least the sum of each
|
|
* queue's min_active.
|
|
*/
|
|
uint32_t zfs_vdev_max_active = 1000;
|
|
|
|
/*
|
|
* Per-queue limits on the number of i/os active to each device. If the
|
|
* number of active i/os is < zfs_vdev_max_active, then the min_active comes
|
|
* into play. We will send min_active from each queue, and then select from
|
|
* queues in the order defined by zio_priority_t.
|
|
*
|
|
* In general, smaller max_active's will lead to lower latency of synchronous
|
|
* operations. Larger max_active's may lead to higher overall throughput,
|
|
* depending on underlying storage.
|
|
*
|
|
* The ratio of the queues' max_actives determines the balance of performance
|
|
* between reads, writes, and scrubs. E.g., increasing
|
|
* zfs_vdev_scrub_max_active will cause the scrub or resilver to complete
|
|
* more quickly, but reads and writes to have higher latency and lower
|
|
* throughput.
|
|
*/
|
|
uint32_t zfs_vdev_sync_read_min_active = 10;
|
|
uint32_t zfs_vdev_sync_read_max_active = 10;
|
|
uint32_t zfs_vdev_sync_write_min_active = 10;
|
|
uint32_t zfs_vdev_sync_write_max_active = 10;
|
|
uint32_t zfs_vdev_async_read_min_active = 1;
|
|
uint32_t zfs_vdev_async_read_max_active = 3;
|
|
uint32_t zfs_vdev_async_write_min_active = 2;
|
|
uint32_t zfs_vdev_async_write_max_active = 10;
|
|
uint32_t zfs_vdev_scrub_min_active = 1;
|
|
uint32_t zfs_vdev_scrub_max_active = 2;
|
|
uint32_t zfs_vdev_removal_min_active = 1;
|
|
uint32_t zfs_vdev_removal_max_active = 2;
|
|
uint32_t zfs_vdev_initializing_min_active = 1;
|
|
uint32_t zfs_vdev_initializing_max_active = 1;
|
|
uint32_t zfs_vdev_trim_min_active = 1;
|
|
uint32_t zfs_vdev_trim_max_active = 2;
|
|
|
|
/*
|
|
* When the pool has less than zfs_vdev_async_write_active_min_dirty_percent
|
|
* dirty data, use zfs_vdev_async_write_min_active. When it has more than
|
|
* zfs_vdev_async_write_active_max_dirty_percent, use
|
|
* zfs_vdev_async_write_max_active. The value is linearly interpolated
|
|
* between min and max.
|
|
*/
|
|
int zfs_vdev_async_write_active_min_dirty_percent = 30;
|
|
int zfs_vdev_async_write_active_max_dirty_percent = 60;
|
|
|
|
/*
|
|
* To reduce IOPs, we aggregate small adjacent I/Os into one large I/O.
|
|
* For read I/Os, we also aggregate across small adjacency gaps; for writes
|
|
* we include spans of optional I/Os to aid aggregation at the disk even when
|
|
* they aren't able to help us aggregate at this level.
|
|
*/
|
|
int zfs_vdev_aggregation_limit = 1 << 20;
|
|
int zfs_vdev_aggregation_limit_non_rotating = SPA_OLD_MAXBLOCKSIZE;
|
|
int zfs_vdev_read_gap_limit = 32 << 10;
|
|
int zfs_vdev_write_gap_limit = 4 << 10;
|
|
|
|
/*
|
|
* Define the queue depth percentage for each top-level. This percentage is
|
|
* used in conjunction with zfs_vdev_async_max_active to determine how many
|
|
* allocations a specific top-level vdev should handle. Once the queue depth
|
|
* reaches zfs_vdev_queue_depth_pct * zfs_vdev_async_write_max_active / 100
|
|
* then allocator will stop allocating blocks on that top-level device.
|
|
* The default kernel setting is 1000% which will yield 100 allocations per
|
|
* device. For userland testing, the default setting is 300% which equates
|
|
* to 30 allocations per device.
|
|
*/
|
|
#ifdef _KERNEL
|
|
int zfs_vdev_queue_depth_pct = 1000;
|
|
#else
|
|
int zfs_vdev_queue_depth_pct = 300;
|
|
#endif
|
|
|
|
/*
|
|
* When performing allocations for a given metaslab, we want to make sure that
|
|
* there are enough IOs to aggregate together to improve throughput. We want to
|
|
* ensure that there are at least 128k worth of IOs that can be aggregated, and
|
|
* we assume that the average allocation size is 4k, so we need the queue depth
|
|
* to be 32 per allocator to get good aggregation of sequential writes.
|
|
*/
|
|
int zfs_vdev_def_queue_depth = 32;
|
|
|
|
/*
|
|
* Allow TRIM I/Os to be aggregated. This should normally not be needed since
|
|
* TRIM I/O for extents up to zfs_trim_extent_bytes_max (128M) can be submitted
|
|
* by the TRIM code in zfs_trim.c.
|
|
*/
|
|
int zfs_vdev_aggregate_trim = 0;
|
|
|
|
int
|
|
vdev_queue_offset_compare(const void *x1, const void *x2)
|
|
{
|
|
const zio_t *z1 = (const zio_t *)x1;
|
|
const zio_t *z2 = (const zio_t *)x2;
|
|
|
|
int cmp = TREE_CMP(z1->io_offset, z2->io_offset);
|
|
|
|
if (likely(cmp))
|
|
return (cmp);
|
|
|
|
return (TREE_PCMP(z1, z2));
|
|
}
|
|
|
|
static inline avl_tree_t *
|
|
vdev_queue_class_tree(vdev_queue_t *vq, zio_priority_t p)
|
|
{
|
|
return (&vq->vq_class[p].vqc_queued_tree);
|
|
}
|
|
|
|
static inline avl_tree_t *
|
|
vdev_queue_type_tree(vdev_queue_t *vq, zio_type_t t)
|
|
{
|
|
ASSERT(t == ZIO_TYPE_READ || t == ZIO_TYPE_WRITE || t == ZIO_TYPE_TRIM);
|
|
if (t == ZIO_TYPE_READ)
|
|
return (&vq->vq_read_offset_tree);
|
|
else if (t == ZIO_TYPE_WRITE)
|
|
return (&vq->vq_write_offset_tree);
|
|
else
|
|
return (&vq->vq_trim_offset_tree);
|
|
}
|
|
|
|
int
|
|
vdev_queue_timestamp_compare(const void *x1, const void *x2)
|
|
{
|
|
const zio_t *z1 = (const zio_t *)x1;
|
|
const zio_t *z2 = (const zio_t *)x2;
|
|
|
|
int cmp = TREE_CMP(z1->io_timestamp, z2->io_timestamp);
|
|
|
|
if (likely(cmp))
|
|
return (cmp);
|
|
|
|
return (TREE_PCMP(z1, z2));
|
|
}
|
|
|
|
static int
|
|
vdev_queue_class_min_active(zio_priority_t p)
|
|
{
|
|
switch (p) {
|
|
case ZIO_PRIORITY_SYNC_READ:
|
|
return (zfs_vdev_sync_read_min_active);
|
|
case ZIO_PRIORITY_SYNC_WRITE:
|
|
return (zfs_vdev_sync_write_min_active);
|
|
case ZIO_PRIORITY_ASYNC_READ:
|
|
return (zfs_vdev_async_read_min_active);
|
|
case ZIO_PRIORITY_ASYNC_WRITE:
|
|
return (zfs_vdev_async_write_min_active);
|
|
case ZIO_PRIORITY_SCRUB:
|
|
return (zfs_vdev_scrub_min_active);
|
|
case ZIO_PRIORITY_REMOVAL:
|
|
return (zfs_vdev_removal_min_active);
|
|
case ZIO_PRIORITY_INITIALIZING:
|
|
return (zfs_vdev_initializing_min_active);
|
|
case ZIO_PRIORITY_TRIM:
|
|
return (zfs_vdev_trim_min_active);
|
|
default:
|
|
panic("invalid priority %u", p);
|
|
return (0);
|
|
}
|
|
}
|
|
|
|
static int
|
|
vdev_queue_max_async_writes(spa_t *spa)
|
|
{
|
|
int writes;
|
|
uint64_t dirty = 0;
|
|
dsl_pool_t *dp = spa_get_dsl(spa);
|
|
uint64_t min_bytes = zfs_dirty_data_max *
|
|
zfs_vdev_async_write_active_min_dirty_percent / 100;
|
|
uint64_t max_bytes = zfs_dirty_data_max *
|
|
zfs_vdev_async_write_active_max_dirty_percent / 100;
|
|
|
|
/*
|
|
* Async writes may occur before the assignment of the spa's
|
|
* dsl_pool_t if a self-healing zio is issued prior to the
|
|
* completion of dmu_objset_open_impl().
|
|
*/
|
|
if (dp == NULL)
|
|
return (zfs_vdev_async_write_max_active);
|
|
|
|
/*
|
|
* Sync tasks correspond to interactive user actions. To reduce the
|
|
* execution time of those actions we push data out as fast as possible.
|
|
*/
|
|
if (spa_has_pending_synctask(spa))
|
|
return (zfs_vdev_async_write_max_active);
|
|
|
|
dirty = dp->dp_dirty_total;
|
|
if (dirty < min_bytes)
|
|
return (zfs_vdev_async_write_min_active);
|
|
if (dirty > max_bytes)
|
|
return (zfs_vdev_async_write_max_active);
|
|
|
|
/*
|
|
* linear interpolation:
|
|
* slope = (max_writes - min_writes) / (max_bytes - min_bytes)
|
|
* move right by min_bytes
|
|
* move up by min_writes
|
|
*/
|
|
writes = (dirty - min_bytes) *
|
|
(zfs_vdev_async_write_max_active -
|
|
zfs_vdev_async_write_min_active) /
|
|
(max_bytes - min_bytes) +
|
|
zfs_vdev_async_write_min_active;
|
|
ASSERT3U(writes, >=, zfs_vdev_async_write_min_active);
|
|
ASSERT3U(writes, <=, zfs_vdev_async_write_max_active);
|
|
return (writes);
|
|
}
|
|
|
|
static int
|
|
vdev_queue_class_max_active(spa_t *spa, zio_priority_t p)
|
|
{
|
|
switch (p) {
|
|
case ZIO_PRIORITY_SYNC_READ:
|
|
return (zfs_vdev_sync_read_max_active);
|
|
case ZIO_PRIORITY_SYNC_WRITE:
|
|
return (zfs_vdev_sync_write_max_active);
|
|
case ZIO_PRIORITY_ASYNC_READ:
|
|
return (zfs_vdev_async_read_max_active);
|
|
case ZIO_PRIORITY_ASYNC_WRITE:
|
|
return (vdev_queue_max_async_writes(spa));
|
|
case ZIO_PRIORITY_SCRUB:
|
|
return (zfs_vdev_scrub_max_active);
|
|
case ZIO_PRIORITY_REMOVAL:
|
|
return (zfs_vdev_removal_max_active);
|
|
case ZIO_PRIORITY_INITIALIZING:
|
|
return (zfs_vdev_initializing_max_active);
|
|
case ZIO_PRIORITY_TRIM:
|
|
return (zfs_vdev_trim_max_active);
|
|
default:
|
|
panic("invalid priority %u", p);
|
|
return (0);
|
|
}
|
|
}
|
|
|
|
/*
|
|
* Return the i/o class to issue from, or ZIO_PRIORITY_MAX_QUEUEABLE if
|
|
* there is no eligible class.
|
|
*/
|
|
static zio_priority_t
|
|
vdev_queue_class_to_issue(vdev_queue_t *vq)
|
|
{
|
|
spa_t *spa = vq->vq_vdev->vdev_spa;
|
|
zio_priority_t p;
|
|
|
|
if (avl_numnodes(&vq->vq_active_tree) >= zfs_vdev_max_active)
|
|
return (ZIO_PRIORITY_NUM_QUEUEABLE);
|
|
|
|
/* find a queue that has not reached its minimum # outstanding i/os */
|
|
for (p = 0; p < ZIO_PRIORITY_NUM_QUEUEABLE; p++) {
|
|
if (avl_numnodes(vdev_queue_class_tree(vq, p)) > 0 &&
|
|
vq->vq_class[p].vqc_active <
|
|
vdev_queue_class_min_active(p))
|
|
return (p);
|
|
}
|
|
|
|
/*
|
|
* If we haven't found a queue, look for one that hasn't reached its
|
|
* maximum # outstanding i/os.
|
|
*/
|
|
for (p = 0; p < ZIO_PRIORITY_NUM_QUEUEABLE; p++) {
|
|
if (avl_numnodes(vdev_queue_class_tree(vq, p)) > 0 &&
|
|
vq->vq_class[p].vqc_active <
|
|
vdev_queue_class_max_active(spa, p))
|
|
return (p);
|
|
}
|
|
|
|
/* No eligible queued i/os */
|
|
return (ZIO_PRIORITY_NUM_QUEUEABLE);
|
|
}
|
|
|
|
void
|
|
vdev_queue_init(vdev_t *vd)
|
|
{
|
|
vdev_queue_t *vq = &vd->vdev_queue;
|
|
zio_priority_t p;
|
|
|
|
mutex_init(&vq->vq_lock, NULL, MUTEX_DEFAULT, NULL);
|
|
vq->vq_vdev = vd;
|
|
taskq_init_ent(&vd->vdev_queue.vq_io_search.io_tqent);
|
|
|
|
avl_create(&vq->vq_active_tree, vdev_queue_offset_compare,
|
|
sizeof (zio_t), offsetof(struct zio, io_queue_node));
|
|
avl_create(vdev_queue_type_tree(vq, ZIO_TYPE_READ),
|
|
vdev_queue_offset_compare, sizeof (zio_t),
|
|
offsetof(struct zio, io_offset_node));
|
|
avl_create(vdev_queue_type_tree(vq, ZIO_TYPE_WRITE),
|
|
vdev_queue_offset_compare, sizeof (zio_t),
|
|
offsetof(struct zio, io_offset_node));
|
|
avl_create(vdev_queue_type_tree(vq, ZIO_TYPE_TRIM),
|
|
vdev_queue_offset_compare, sizeof (zio_t),
|
|
offsetof(struct zio, io_offset_node));
|
|
|
|
for (p = 0; p < ZIO_PRIORITY_NUM_QUEUEABLE; p++) {
|
|
int (*compfn) (const void *, const void *);
|
|
|
|
/*
|
|
* The synchronous/trim i/o queues are dispatched in FIFO rather
|
|
* than LBA order. This provides more consistent latency for
|
|
* these i/os.
|
|
*/
|
|
if (p == ZIO_PRIORITY_SYNC_READ ||
|
|
p == ZIO_PRIORITY_SYNC_WRITE ||
|
|
p == ZIO_PRIORITY_TRIM) {
|
|
compfn = vdev_queue_timestamp_compare;
|
|
} else {
|
|
compfn = vdev_queue_offset_compare;
|
|
}
|
|
avl_create(vdev_queue_class_tree(vq, p), compfn,
|
|
sizeof (zio_t), offsetof(struct zio, io_queue_node));
|
|
}
|
|
|
|
vq->vq_last_offset = 0;
|
|
}
|
|
|
|
void
|
|
vdev_queue_fini(vdev_t *vd)
|
|
{
|
|
vdev_queue_t *vq = &vd->vdev_queue;
|
|
|
|
for (zio_priority_t p = 0; p < ZIO_PRIORITY_NUM_QUEUEABLE; p++)
|
|
avl_destroy(vdev_queue_class_tree(vq, p));
|
|
avl_destroy(&vq->vq_active_tree);
|
|
avl_destroy(vdev_queue_type_tree(vq, ZIO_TYPE_READ));
|
|
avl_destroy(vdev_queue_type_tree(vq, ZIO_TYPE_WRITE));
|
|
avl_destroy(vdev_queue_type_tree(vq, ZIO_TYPE_TRIM));
|
|
|
|
mutex_destroy(&vq->vq_lock);
|
|
}
|
|
|
|
static void
|
|
vdev_queue_io_add(vdev_queue_t *vq, zio_t *zio)
|
|
{
|
|
spa_t *spa = zio->io_spa;
|
|
spa_history_kstat_t *shk = &spa->spa_stats.io_history;
|
|
|
|
ASSERT3U(zio->io_priority, <, ZIO_PRIORITY_NUM_QUEUEABLE);
|
|
avl_add(vdev_queue_class_tree(vq, zio->io_priority), zio);
|
|
avl_add(vdev_queue_type_tree(vq, zio->io_type), zio);
|
|
|
|
if (shk->kstat != NULL) {
|
|
mutex_enter(&shk->lock);
|
|
kstat_waitq_enter(shk->kstat->ks_data);
|
|
mutex_exit(&shk->lock);
|
|
}
|
|
}
|
|
|
|
static void
|
|
vdev_queue_io_remove(vdev_queue_t *vq, zio_t *zio)
|
|
{
|
|
spa_t *spa = zio->io_spa;
|
|
spa_history_kstat_t *shk = &spa->spa_stats.io_history;
|
|
|
|
ASSERT3U(zio->io_priority, <, ZIO_PRIORITY_NUM_QUEUEABLE);
|
|
avl_remove(vdev_queue_class_tree(vq, zio->io_priority), zio);
|
|
avl_remove(vdev_queue_type_tree(vq, zio->io_type), zio);
|
|
|
|
if (shk->kstat != NULL) {
|
|
mutex_enter(&shk->lock);
|
|
kstat_waitq_exit(shk->kstat->ks_data);
|
|
mutex_exit(&shk->lock);
|
|
}
|
|
}
|
|
|
|
static void
|
|
vdev_queue_pending_add(vdev_queue_t *vq, zio_t *zio)
|
|
{
|
|
spa_t *spa = zio->io_spa;
|
|
spa_history_kstat_t *shk = &spa->spa_stats.io_history;
|
|
|
|
ASSERT(MUTEX_HELD(&vq->vq_lock));
|
|
ASSERT3U(zio->io_priority, <, ZIO_PRIORITY_NUM_QUEUEABLE);
|
|
vq->vq_class[zio->io_priority].vqc_active++;
|
|
avl_add(&vq->vq_active_tree, zio);
|
|
|
|
if (shk->kstat != NULL) {
|
|
mutex_enter(&shk->lock);
|
|
kstat_runq_enter(shk->kstat->ks_data);
|
|
mutex_exit(&shk->lock);
|
|
}
|
|
}
|
|
|
|
static void
|
|
vdev_queue_pending_remove(vdev_queue_t *vq, zio_t *zio)
|
|
{
|
|
spa_t *spa = zio->io_spa;
|
|
spa_history_kstat_t *shk = &spa->spa_stats.io_history;
|
|
|
|
ASSERT(MUTEX_HELD(&vq->vq_lock));
|
|
ASSERT3U(zio->io_priority, <, ZIO_PRIORITY_NUM_QUEUEABLE);
|
|
vq->vq_class[zio->io_priority].vqc_active--;
|
|
avl_remove(&vq->vq_active_tree, zio);
|
|
|
|
if (shk->kstat != NULL) {
|
|
kstat_io_t *ksio = shk->kstat->ks_data;
|
|
|
|
mutex_enter(&shk->lock);
|
|
kstat_runq_exit(ksio);
|
|
if (zio->io_type == ZIO_TYPE_READ) {
|
|
ksio->reads++;
|
|
ksio->nread += zio->io_size;
|
|
} else if (zio->io_type == ZIO_TYPE_WRITE) {
|
|
ksio->writes++;
|
|
ksio->nwritten += zio->io_size;
|
|
}
|
|
mutex_exit(&shk->lock);
|
|
}
|
|
}
|
|
|
|
static void
|
|
vdev_queue_agg_io_done(zio_t *aio)
|
|
{
|
|
if (aio->io_type == ZIO_TYPE_READ) {
|
|
zio_t *pio;
|
|
zio_link_t *zl = NULL;
|
|
while ((pio = zio_walk_parents(aio, &zl)) != NULL) {
|
|
abd_copy_off(pio->io_abd, aio->io_abd,
|
|
0, pio->io_offset - aio->io_offset, pio->io_size);
|
|
}
|
|
}
|
|
|
|
abd_free(aio->io_abd);
|
|
}
|
|
|
|
/*
|
|
* Compute the range spanned by two i/os, which is the endpoint of the last
|
|
* (lio->io_offset + lio->io_size) minus start of the first (fio->io_offset).
|
|
* Conveniently, the gap between fio and lio is given by -IO_SPAN(lio, fio);
|
|
* thus fio and lio are adjacent if and only if IO_SPAN(lio, fio) == 0.
|
|
*/
|
|
#define IO_SPAN(fio, lio) ((lio)->io_offset + (lio)->io_size - (fio)->io_offset)
|
|
#define IO_GAP(fio, lio) (-IO_SPAN(lio, fio))
|
|
|
|
static zio_t *
|
|
vdev_queue_aggregate(vdev_queue_t *vq, zio_t *zio)
|
|
{
|
|
zio_t *first, *last, *aio, *dio, *mandatory, *nio;
|
|
zio_link_t *zl = NULL;
|
|
uint64_t maxgap = 0;
|
|
uint64_t size;
|
|
uint64_t limit;
|
|
int maxblocksize;
|
|
boolean_t stretch = B_FALSE;
|
|
avl_tree_t *t = vdev_queue_type_tree(vq, zio->io_type);
|
|
enum zio_flag flags = zio->io_flags & ZIO_FLAG_AGG_INHERIT;
|
|
abd_t *abd;
|
|
|
|
maxblocksize = spa_maxblocksize(vq->vq_vdev->vdev_spa);
|
|
if (vq->vq_vdev->vdev_nonrot)
|
|
limit = zfs_vdev_aggregation_limit_non_rotating;
|
|
else
|
|
limit = zfs_vdev_aggregation_limit;
|
|
limit = MAX(MIN(limit, maxblocksize), 0);
|
|
|
|
if (zio->io_flags & ZIO_FLAG_DONT_AGGREGATE || limit == 0)
|
|
return (NULL);
|
|
|
|
/*
|
|
* While TRIM commands could be aggregated based on offset this
|
|
* behavior is disabled until it's determined to be beneficial.
|
|
*/
|
|
if (zio->io_type == ZIO_TYPE_TRIM && !zfs_vdev_aggregate_trim)
|
|
return (NULL);
|
|
|
|
first = last = zio;
|
|
|
|
if (zio->io_type == ZIO_TYPE_READ)
|
|
maxgap = zfs_vdev_read_gap_limit;
|
|
|
|
/*
|
|
* We can aggregate I/Os that are sufficiently adjacent and of
|
|
* the same flavor, as expressed by the AGG_INHERIT flags.
|
|
* The latter requirement is necessary so that certain
|
|
* attributes of the I/O, such as whether it's a normal I/O
|
|
* or a scrub/resilver, can be preserved in the aggregate.
|
|
* We can include optional I/Os, but don't allow them
|
|
* to begin a range as they add no benefit in that situation.
|
|
*/
|
|
|
|
/*
|
|
* We keep track of the last non-optional I/O.
|
|
*/
|
|
mandatory = (first->io_flags & ZIO_FLAG_OPTIONAL) ? NULL : first;
|
|
|
|
/*
|
|
* Walk backwards through sufficiently contiguous I/Os
|
|
* recording the last non-optional I/O.
|
|
*/
|
|
while ((dio = AVL_PREV(t, first)) != NULL &&
|
|
(dio->io_flags & ZIO_FLAG_AGG_INHERIT) == flags &&
|
|
IO_SPAN(dio, last) <= limit &&
|
|
IO_GAP(dio, first) <= maxgap &&
|
|
dio->io_type == zio->io_type) {
|
|
first = dio;
|
|
if (mandatory == NULL && !(first->io_flags & ZIO_FLAG_OPTIONAL))
|
|
mandatory = first;
|
|
}
|
|
|
|
/*
|
|
* Skip any initial optional I/Os.
|
|
*/
|
|
while ((first->io_flags & ZIO_FLAG_OPTIONAL) && first != last) {
|
|
first = AVL_NEXT(t, first);
|
|
ASSERT(first != NULL);
|
|
}
|
|
|
|
|
|
/*
|
|
* Walk forward through sufficiently contiguous I/Os.
|
|
* The aggregation limit does not apply to optional i/os, so that
|
|
* we can issue contiguous writes even if they are larger than the
|
|
* aggregation limit.
|
|
*/
|
|
while ((dio = AVL_NEXT(t, last)) != NULL &&
|
|
(dio->io_flags & ZIO_FLAG_AGG_INHERIT) == flags &&
|
|
(IO_SPAN(first, dio) <= limit ||
|
|
(dio->io_flags & ZIO_FLAG_OPTIONAL)) &&
|
|
IO_SPAN(first, dio) <= maxblocksize &&
|
|
IO_GAP(last, dio) <= maxgap &&
|
|
dio->io_type == zio->io_type) {
|
|
last = dio;
|
|
if (!(last->io_flags & ZIO_FLAG_OPTIONAL))
|
|
mandatory = last;
|
|
}
|
|
|
|
/*
|
|
* Now that we've established the range of the I/O aggregation
|
|
* we must decide what to do with trailing optional I/Os.
|
|
* For reads, there's nothing to do. While we are unable to
|
|
* aggregate further, it's possible that a trailing optional
|
|
* I/O would allow the underlying device to aggregate with
|
|
* subsequent I/Os. We must therefore determine if the next
|
|
* non-optional I/O is close enough to make aggregation
|
|
* worthwhile.
|
|
*/
|
|
if (zio->io_type == ZIO_TYPE_WRITE && mandatory != NULL) {
|
|
zio_t *nio = last;
|
|
while ((dio = AVL_NEXT(t, nio)) != NULL &&
|
|
IO_GAP(nio, dio) == 0 &&
|
|
IO_GAP(mandatory, dio) <= zfs_vdev_write_gap_limit) {
|
|
nio = dio;
|
|
if (!(nio->io_flags & ZIO_FLAG_OPTIONAL)) {
|
|
stretch = B_TRUE;
|
|
break;
|
|
}
|
|
}
|
|
}
|
|
|
|
if (stretch) {
|
|
/*
|
|
* We are going to include an optional io in our aggregated
|
|
* span, thus closing the write gap. Only mandatory i/os can
|
|
* start aggregated spans, so make sure that the next i/o
|
|
* after our span is mandatory.
|
|
*/
|
|
dio = AVL_NEXT(t, last);
|
|
dio->io_flags &= ~ZIO_FLAG_OPTIONAL;
|
|
} else {
|
|
/* do not include the optional i/o */
|
|
while (last != mandatory && last != first) {
|
|
ASSERT(last->io_flags & ZIO_FLAG_OPTIONAL);
|
|
last = AVL_PREV(t, last);
|
|
ASSERT(last != NULL);
|
|
}
|
|
}
|
|
|
|
if (first == last)
|
|
return (NULL);
|
|
|
|
size = IO_SPAN(first, last);
|
|
ASSERT3U(size, <=, maxblocksize);
|
|
|
|
abd = abd_alloc_for_io(size, B_TRUE);
|
|
if (abd == NULL)
|
|
return (NULL);
|
|
|
|
aio = zio_vdev_delegated_io(first->io_vd, first->io_offset,
|
|
abd, size, first->io_type, zio->io_priority,
|
|
flags | ZIO_FLAG_DONT_CACHE | ZIO_FLAG_DONT_QUEUE,
|
|
vdev_queue_agg_io_done, NULL);
|
|
aio->io_timestamp = first->io_timestamp;
|
|
|
|
nio = first;
|
|
do {
|
|
dio = nio;
|
|
nio = AVL_NEXT(t, dio);
|
|
zio_add_child(dio, aio);
|
|
vdev_queue_io_remove(vq, dio);
|
|
} while (dio != last);
|
|
|
|
/*
|
|
* We need to drop the vdev queue's lock during zio_execute() to
|
|
* avoid a deadlock that we could encounter due to lock order
|
|
* reversal between vq_lock and io_lock in zio_change_priority().
|
|
* Use the dropped lock to do memory copy without congestion.
|
|
*/
|
|
mutex_exit(&vq->vq_lock);
|
|
while ((dio = zio_walk_parents(aio, &zl)) != NULL) {
|
|
ASSERT3U(dio->io_type, ==, aio->io_type);
|
|
|
|
if (dio->io_flags & ZIO_FLAG_NODATA) {
|
|
ASSERT3U(dio->io_type, ==, ZIO_TYPE_WRITE);
|
|
abd_zero_off(aio->io_abd,
|
|
dio->io_offset - aio->io_offset, dio->io_size);
|
|
} else if (dio->io_type == ZIO_TYPE_WRITE) {
|
|
abd_copy_off(aio->io_abd, dio->io_abd,
|
|
dio->io_offset - aio->io_offset, 0, dio->io_size);
|
|
}
|
|
|
|
zio_vdev_io_bypass(dio);
|
|
zio_execute(dio);
|
|
}
|
|
mutex_enter(&vq->vq_lock);
|
|
|
|
return (aio);
|
|
}
|
|
|
|
static zio_t *
|
|
vdev_queue_io_to_issue(vdev_queue_t *vq)
|
|
{
|
|
zio_t *zio, *aio;
|
|
zio_priority_t p;
|
|
avl_index_t idx;
|
|
avl_tree_t *tree;
|
|
|
|
again:
|
|
ASSERT(MUTEX_HELD(&vq->vq_lock));
|
|
|
|
p = vdev_queue_class_to_issue(vq);
|
|
|
|
if (p == ZIO_PRIORITY_NUM_QUEUEABLE) {
|
|
/* No eligible queued i/os */
|
|
return (NULL);
|
|
}
|
|
|
|
/*
|
|
* For LBA-ordered queues (async / scrub / initializing), issue the
|
|
* i/o which follows the most recently issued i/o in LBA (offset) order.
|
|
*
|
|
* For FIFO queues (sync/trim), issue the i/o with the lowest timestamp.
|
|
*/
|
|
tree = vdev_queue_class_tree(vq, p);
|
|
vq->vq_io_search.io_timestamp = 0;
|
|
vq->vq_io_search.io_offset = vq->vq_last_offset - 1;
|
|
VERIFY3P(avl_find(tree, &vq->vq_io_search, &idx), ==, NULL);
|
|
zio = avl_nearest(tree, idx, AVL_AFTER);
|
|
if (zio == NULL)
|
|
zio = avl_first(tree);
|
|
ASSERT3U(zio->io_priority, ==, p);
|
|
|
|
aio = vdev_queue_aggregate(vq, zio);
|
|
if (aio != NULL)
|
|
zio = aio;
|
|
else
|
|
vdev_queue_io_remove(vq, zio);
|
|
|
|
/*
|
|
* If the I/O is or was optional and therefore has no data, we need to
|
|
* simply discard it. We need to drop the vdev queue's lock to avoid a
|
|
* deadlock that we could encounter since this I/O will complete
|
|
* immediately.
|
|
*/
|
|
if (zio->io_flags & ZIO_FLAG_NODATA) {
|
|
mutex_exit(&vq->vq_lock);
|
|
zio_vdev_io_bypass(zio);
|
|
zio_execute(zio);
|
|
mutex_enter(&vq->vq_lock);
|
|
goto again;
|
|
}
|
|
|
|
vdev_queue_pending_add(vq, zio);
|
|
vq->vq_last_offset = zio->io_offset + zio->io_size;
|
|
|
|
return (zio);
|
|
}
|
|
|
|
zio_t *
|
|
vdev_queue_io(zio_t *zio)
|
|
{
|
|
vdev_queue_t *vq = &zio->io_vd->vdev_queue;
|
|
zio_t *nio;
|
|
|
|
if (zio->io_flags & ZIO_FLAG_DONT_QUEUE)
|
|
return (zio);
|
|
|
|
/*
|
|
* Children i/os inherent their parent's priority, which might
|
|
* not match the child's i/o type. Fix it up here.
|
|
*/
|
|
if (zio->io_type == ZIO_TYPE_READ) {
|
|
ASSERT(zio->io_priority != ZIO_PRIORITY_TRIM);
|
|
|
|
if (zio->io_priority != ZIO_PRIORITY_SYNC_READ &&
|
|
zio->io_priority != ZIO_PRIORITY_ASYNC_READ &&
|
|
zio->io_priority != ZIO_PRIORITY_SCRUB &&
|
|
zio->io_priority != ZIO_PRIORITY_REMOVAL &&
|
|
zio->io_priority != ZIO_PRIORITY_INITIALIZING) {
|
|
zio->io_priority = ZIO_PRIORITY_ASYNC_READ;
|
|
}
|
|
} else if (zio->io_type == ZIO_TYPE_WRITE) {
|
|
ASSERT(zio->io_priority != ZIO_PRIORITY_TRIM);
|
|
|
|
if (zio->io_priority != ZIO_PRIORITY_SYNC_WRITE &&
|
|
zio->io_priority != ZIO_PRIORITY_ASYNC_WRITE &&
|
|
zio->io_priority != ZIO_PRIORITY_REMOVAL &&
|
|
zio->io_priority != ZIO_PRIORITY_INITIALIZING) {
|
|
zio->io_priority = ZIO_PRIORITY_ASYNC_WRITE;
|
|
}
|
|
} else {
|
|
ASSERT(zio->io_type == ZIO_TYPE_TRIM);
|
|
ASSERT(zio->io_priority == ZIO_PRIORITY_TRIM);
|
|
}
|
|
|
|
zio->io_flags |= ZIO_FLAG_DONT_CACHE | ZIO_FLAG_DONT_QUEUE;
|
|
|
|
mutex_enter(&vq->vq_lock);
|
|
zio->io_timestamp = gethrtime();
|
|
vdev_queue_io_add(vq, zio);
|
|
nio = vdev_queue_io_to_issue(vq);
|
|
mutex_exit(&vq->vq_lock);
|
|
|
|
if (nio == NULL)
|
|
return (NULL);
|
|
|
|
if (nio->io_done == vdev_queue_agg_io_done) {
|
|
zio_nowait(nio);
|
|
return (NULL);
|
|
}
|
|
|
|
return (nio);
|
|
}
|
|
|
|
void
|
|
vdev_queue_io_done(zio_t *zio)
|
|
{
|
|
vdev_queue_t *vq = &zio->io_vd->vdev_queue;
|
|
zio_t *nio;
|
|
|
|
mutex_enter(&vq->vq_lock);
|
|
|
|
vdev_queue_pending_remove(vq, zio);
|
|
|
|
zio->io_delta = gethrtime() - zio->io_timestamp;
|
|
vq->vq_io_complete_ts = gethrtime();
|
|
vq->vq_io_delta_ts = vq->vq_io_complete_ts - zio->io_timestamp;
|
|
|
|
while ((nio = vdev_queue_io_to_issue(vq)) != NULL) {
|
|
mutex_exit(&vq->vq_lock);
|
|
if (nio->io_done == vdev_queue_agg_io_done) {
|
|
zio_nowait(nio);
|
|
} else {
|
|
zio_vdev_io_reissue(nio);
|
|
zio_execute(nio);
|
|
}
|
|
mutex_enter(&vq->vq_lock);
|
|
}
|
|
|
|
mutex_exit(&vq->vq_lock);
|
|
}
|
|
|
|
void
|
|
vdev_queue_change_io_priority(zio_t *zio, zio_priority_t priority)
|
|
{
|
|
vdev_queue_t *vq = &zio->io_vd->vdev_queue;
|
|
avl_tree_t *tree;
|
|
|
|
/*
|
|
* ZIO_PRIORITY_NOW is used by the vdev cache code and the aggregate zio
|
|
* code to issue IOs without adding them to the vdev queue. In this
|
|
* case, the zio is already going to be issued as quickly as possible
|
|
* and so it doesn't need any reprioritization to help.
|
|
*/
|
|
if (zio->io_priority == ZIO_PRIORITY_NOW)
|
|
return;
|
|
|
|
ASSERT3U(zio->io_priority, <, ZIO_PRIORITY_NUM_QUEUEABLE);
|
|
ASSERT3U(priority, <, ZIO_PRIORITY_NUM_QUEUEABLE);
|
|
|
|
if (zio->io_type == ZIO_TYPE_READ) {
|
|
if (priority != ZIO_PRIORITY_SYNC_READ &&
|
|
priority != ZIO_PRIORITY_ASYNC_READ &&
|
|
priority != ZIO_PRIORITY_SCRUB)
|
|
priority = ZIO_PRIORITY_ASYNC_READ;
|
|
} else {
|
|
ASSERT(zio->io_type == ZIO_TYPE_WRITE);
|
|
if (priority != ZIO_PRIORITY_SYNC_WRITE &&
|
|
priority != ZIO_PRIORITY_ASYNC_WRITE)
|
|
priority = ZIO_PRIORITY_ASYNC_WRITE;
|
|
}
|
|
|
|
mutex_enter(&vq->vq_lock);
|
|
|
|
/*
|
|
* If the zio is in none of the queues we can simply change
|
|
* the priority. If the zio is waiting to be submitted we must
|
|
* remove it from the queue and re-insert it with the new priority.
|
|
* Otherwise, the zio is currently active and we cannot change its
|
|
* priority.
|
|
*/
|
|
tree = vdev_queue_class_tree(vq, zio->io_priority);
|
|
if (avl_find(tree, zio, NULL) == zio) {
|
|
avl_remove(vdev_queue_class_tree(vq, zio->io_priority), zio);
|
|
zio->io_priority = priority;
|
|
avl_add(vdev_queue_class_tree(vq, zio->io_priority), zio);
|
|
} else if (avl_find(&vq->vq_active_tree, zio, NULL) != zio) {
|
|
zio->io_priority = priority;
|
|
}
|
|
|
|
mutex_exit(&vq->vq_lock);
|
|
}
|
|
|
|
/*
|
|
* As these two methods are only used for load calculations we're not
|
|
* concerned if we get an incorrect value on 32bit platforms due to lack of
|
|
* vq_lock mutex use here, instead we prefer to keep it lock free for
|
|
* performance.
|
|
*/
|
|
int
|
|
vdev_queue_length(vdev_t *vd)
|
|
{
|
|
return (avl_numnodes(&vd->vdev_queue.vq_active_tree));
|
|
}
|
|
|
|
uint64_t
|
|
vdev_queue_last_offset(vdev_t *vd)
|
|
{
|
|
return (vd->vdev_queue.vq_last_offset);
|
|
}
|
|
|
|
/* BEGIN CSTYLED */
|
|
ZFS_MODULE_PARAM(zfs_vdev, zfs_vdev_, aggregation_limit, INT, ZMOD_RW,
|
|
"Max vdev I/O aggregation size");
|
|
|
|
ZFS_MODULE_PARAM(zfs_vdev, zfs_vdev_, aggregation_limit_non_rotating, INT, ZMOD_RW,
|
|
"Max vdev I/O aggregation size for non-rotating media");
|
|
|
|
ZFS_MODULE_PARAM(zfs_vdev, zfs_vdev_, aggregate_trim, INT, ZMOD_RW,
|
|
"Allow TRIM I/O to be aggregated");
|
|
|
|
ZFS_MODULE_PARAM(zfs_vdev, zfs_vdev_, read_gap_limit, INT, ZMOD_RW,
|
|
"Aggregate read I/O over gap");
|
|
|
|
ZFS_MODULE_PARAM(zfs_vdev, zfs_vdev_, write_gap_limit, INT, ZMOD_RW,
|
|
"Aggregate write I/O over gap");
|
|
|
|
ZFS_MODULE_PARAM(zfs_vdev, zfs_vdev_, max_active, INT, ZMOD_RW,
|
|
"Maximum number of active I/Os per vdev");
|
|
|
|
ZFS_MODULE_PARAM(zfs_vdev, zfs_vdev_, async_write_active_max_dirty_percent, INT, ZMOD_RW,
|
|
"Async write concurrency max threshold");
|
|
|
|
ZFS_MODULE_PARAM(zfs_vdev, zfs_vdev_, async_write_active_min_dirty_percent, INT, ZMOD_RW,
|
|
"Async write concurrency min threshold");
|
|
|
|
ZFS_MODULE_PARAM(zfs_vdev, zfs_vdev_, async_read_max_active, INT, ZMOD_RW,
|
|
"Max active async read I/Os per vdev");
|
|
|
|
ZFS_MODULE_PARAM(zfs_vdev, zfs_vdev_, async_read_min_active, INT, ZMOD_RW,
|
|
"Min active async read I/Os per vdev");
|
|
|
|
ZFS_MODULE_PARAM(zfs_vdev, zfs_vdev_, async_write_max_active, INT, ZMOD_RW,
|
|
"Max active async write I/Os per vdev");
|
|
|
|
ZFS_MODULE_PARAM(zfs_vdev, zfs_vdev_, async_write_min_active, INT, ZMOD_RW,
|
|
"Min active async write I/Os per vdev");
|
|
|
|
ZFS_MODULE_PARAM(zfs_vdev, zfs_vdev_, initializing_max_active, INT, ZMOD_RW,
|
|
"Max active initializing I/Os per vdev");
|
|
|
|
ZFS_MODULE_PARAM(zfs_vdev, zfs_vdev_, initializing_min_active, INT, ZMOD_RW,
|
|
"Min active initializing I/Os per vdev");
|
|
|
|
ZFS_MODULE_PARAM(zfs_vdev, zfs_vdev_, removal_max_active, INT, ZMOD_RW,
|
|
"Max active removal I/Os per vdev");
|
|
|
|
ZFS_MODULE_PARAM(zfs_vdev, zfs_vdev_, removal_min_active, INT, ZMOD_RW,
|
|
"Min active removal I/Os per vdev");
|
|
|
|
ZFS_MODULE_PARAM(zfs_vdev, zfs_vdev_, scrub_max_active, INT, ZMOD_RW,
|
|
"Max active scrub I/Os per vdev");
|
|
|
|
ZFS_MODULE_PARAM(zfs_vdev, zfs_vdev_, scrub_min_active, INT, ZMOD_RW,
|
|
"Min active scrub I/Os per vdev");
|
|
|
|
ZFS_MODULE_PARAM(zfs_vdev, zfs_vdev_, sync_read_max_active, INT, ZMOD_RW,
|
|
"Max active sync read I/Os per vdev");
|
|
|
|
ZFS_MODULE_PARAM(zfs_vdev, zfs_vdev_, sync_read_min_active, INT, ZMOD_RW,
|
|
"Min active sync read I/Os per vdev");
|
|
|
|
ZFS_MODULE_PARAM(zfs_vdev, zfs_vdev_, sync_write_max_active, INT, ZMOD_RW,
|
|
"Max active sync write I/Os per vdev");
|
|
|
|
ZFS_MODULE_PARAM(zfs_vdev, zfs_vdev_, sync_write_min_active, INT, ZMOD_RW,
|
|
"Min active sync write I/Os per vdev");
|
|
|
|
ZFS_MODULE_PARAM(zfs_vdev, zfs_vdev_, trim_max_active, INT, ZMOD_RW,
|
|
"Max active trim/discard I/Os per vdev");
|
|
|
|
ZFS_MODULE_PARAM(zfs_vdev, zfs_vdev_, trim_min_active, INT, ZMOD_RW,
|
|
"Min active trim/discard I/Os per vdev");
|
|
|
|
ZFS_MODULE_PARAM(zfs_vdev, zfs_vdev_, queue_depth_pct, INT, ZMOD_RW,
|
|
"Queue depth percentage for each top-level vdev");
|
|
/* END CSTYLED */
|