mirror of
https://git.proxmox.com/git/mirror_zfs.git
synced 2025-01-18 14:07:10 +03:00
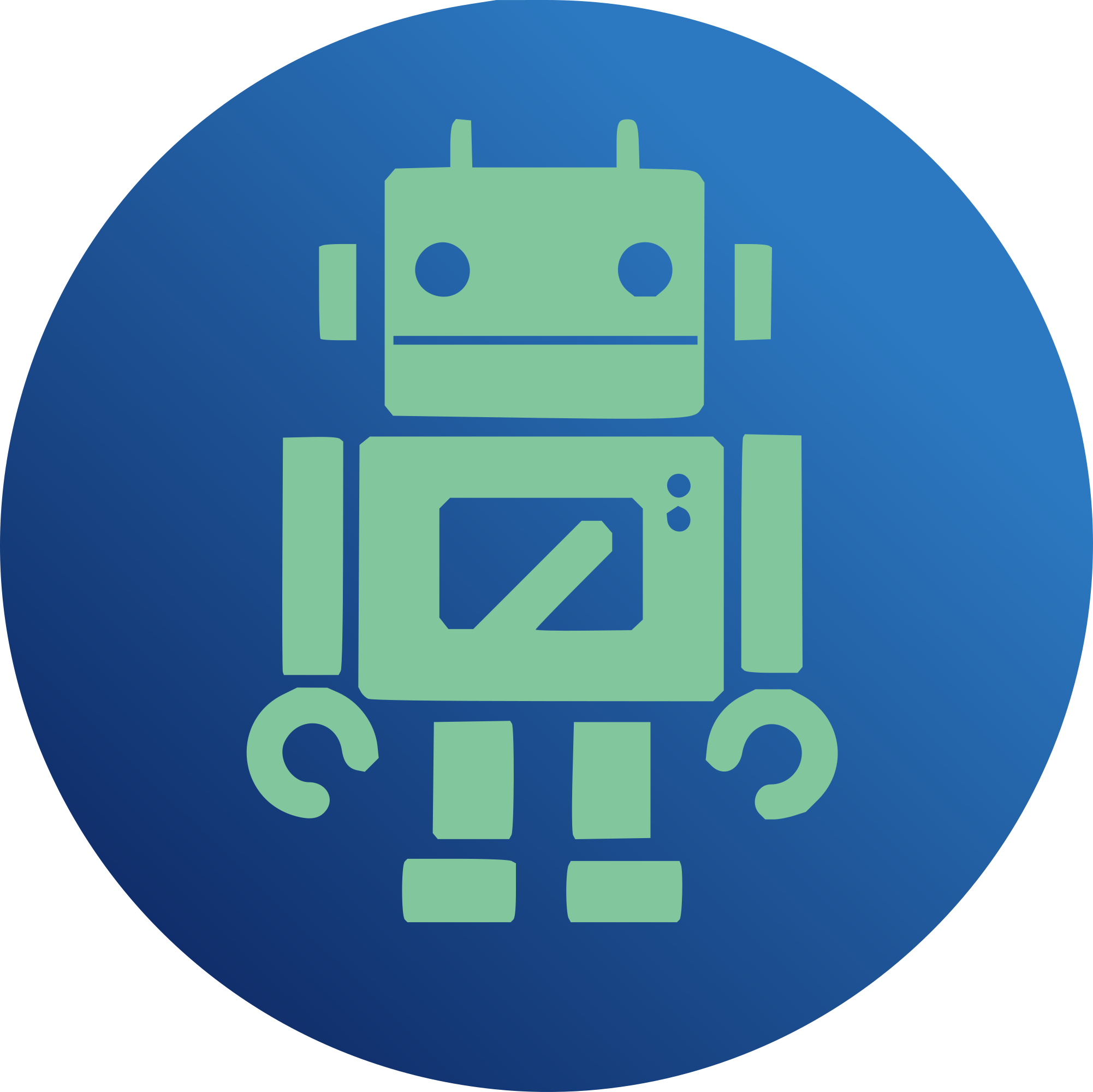
This patch implements a new tree structure for ZFS, and uses it to store range trees more efficiently. The new structure is approximately a B-tree, though there are some small differences from the usual characterizations. The tree has core nodes and leaf nodes; each contain data elements, which the elements in the core nodes acting as separators between its children. The difference between core and leaf nodes is that the core nodes have an array of children, while leaf nodes don't. Every node in the tree may be only partially full; in most cases, they are all at least 50% full (in terms of element count) except for the root node, which can be less full. Underfull nodes will steal from their neighbors or merge to remain full enough, while overfull nodes will split in two. The data elements are contained in tree-controlled buffers; they are copied into these on insertion, and overwritten on deletion. This means that the elements are not independently allocated, which reduces overhead, but also means they can't be shared between trees (and also that pointers to them are only valid until a side-effectful tree operation occurs). The overhead varies based on how dense the tree is, but is usually on the order of about 50% of the element size; the per-node overheads are very small, and so don't make a significant difference. The trees can accept arbitrary records; they accept a size and a comparator to allow them to be used for a variety of purposes. The new trees replace the AVL trees used in the range trees today. Currently, the range_seg_t structure contains three 8 byte integers of payload and two 24 byte avl_tree_node_ts to handle its storage in both an offset-sorted tree and a size-sorted tree (total size: 64 bytes). In the new model, the range seg structures are usually two 4 byte integers, but a separate one needs to exist for the size-sorted and offset-sorted tree. Between the raw size, the 50% overhead, and the double storage, the new btrees are expected to use 8*1.5*2 = 24 bytes per record, or 33.3% as much memory as the AVL trees (this is for the purposes of storing metaslab range trees; for other purposes, like scrubs, they use ~50% as much memory). We reduced the size of the payload in the range segments by teaching range trees about starting offsets and shifts; since metaslabs have a fixed starting offset, and they all operate in terms of disk sectors, we can store the ranges using 4-byte integers as long as the size of the metaslab divided by the sector size is less than 2^32. For 512-byte sectors, this is a 2^41 (or 2TB) metaslab, which with the default settings corresponds to a 256PB disk. 4k sector disks can handle metaslabs up to 2^46 bytes, or 2^63 byte disks. Since we do not anticipate disks of this size in the near future, there should be almost no cases where metaslabs need 64-byte integers to store their ranges. We do still have the capability to store 64-byte integer ranges to account for cases where we are storing per-vdev (or per-dnode) trees, which could reasonably go above the limits discussed. We also do not store fill information in the compact version of the node, since it is only used for sorted scrub. We also optimized the metaslab loading process in various other ways to offset some inefficiencies in the btree model. While individual operations (find, insert, remove_from) are faster for the btree than they are for the avl tree, remove usually requires a find operation, while in the AVL tree model the element itself suffices. Some clever changes actually caused an overall speedup in metaslab loading; we use approximately 40% less cpu to load metaslabs in our tests on Illumos. Another memory and performance optimization was achieved by changing what is stored in the size-sorted trees. When a disk is heavily fragmented, the df algorithm used by default in ZFS will almost always find a number of small regions in its initial cursor-based search; it will usually only fall back to the size-sorted tree to find larger regions. If we increase the size of the cursor-based search slightly, and don't store segments that are smaller than a tunable size floor in the size-sorted tree, we can further cut memory usage down to below 20% of what the AVL trees store. This also results in further reductions in CPU time spent loading metaslabs. The 16KiB size floor was chosen because it results in substantial memory usage reduction while not usually resulting in situations where we can't find an appropriate chunk with the cursor and are forced to use an oversized chunk from the size-sorted tree. In addition, even if we do have to use an oversized chunk from the size-sorted tree, the chunk would be too small to use for ZIL allocations, so it isn't as big of a loss as it might otherwise be. And often, more small allocations will follow the initial one, and the cursor search will now find the remainder of the chunk we didn't use all of and use it for subsequent allocations. Practical testing has shown little or no change in fragmentation as a result of this change. If the size-sorted tree becomes empty while the offset sorted one still has entries, it will load all the entries from the offset sorted tree and disregard the size floor until it is unloaded again. This operation occurs rarely with the default setting, only on incredibly thoroughly fragmented pools. There are some other small changes to zdb to teach it to handle btrees, but nothing major. Reviewed-by: George Wilson <gwilson@delphix.com> Reviewed-by: Matt Ahrens <matt@delphix.com> Reviewed by: Sebastien Roy seb@delphix.com Reviewed-by: Igor Kozhukhov <igor@dilos.org> Reviewed-by: Brian Behlendorf <behlendorf1@llnl.gov> Signed-off-by: Paul Dagnelie <pcd@delphix.com> Closes #9181
556 lines
21 KiB
C
556 lines
21 KiB
C
/*
|
|
* CDDL HEADER START
|
|
*
|
|
* The contents of this file are subject to the terms of the
|
|
* Common Development and Distribution License (the "License").
|
|
* You may not use this file except in compliance with the License.
|
|
*
|
|
* You can obtain a copy of the license at usr/src/OPENSOLARIS.LICENSE
|
|
* or http://www.opensolaris.org/os/licensing.
|
|
* See the License for the specific language governing permissions
|
|
* and limitations under the License.
|
|
*
|
|
* When distributing Covered Code, include this CDDL HEADER in each
|
|
* file and include the License file at usr/src/OPENSOLARIS.LICENSE.
|
|
* If applicable, add the following below this CDDL HEADER, with the
|
|
* fields enclosed by brackets "[]" replaced with your own identifying
|
|
* information: Portions Copyright [yyyy] [name of copyright owner]
|
|
*
|
|
* CDDL HEADER END
|
|
*/
|
|
/*
|
|
* Copyright 2009 Sun Microsystems, Inc. All rights reserved.
|
|
* Use is subject to license terms.
|
|
*/
|
|
|
|
/*
|
|
* Copyright (c) 2011, 2019 by Delphix. All rights reserved.
|
|
*/
|
|
|
|
#ifndef _SYS_METASLAB_IMPL_H
|
|
#define _SYS_METASLAB_IMPL_H
|
|
|
|
#include <sys/metaslab.h>
|
|
#include <sys/space_map.h>
|
|
#include <sys/range_tree.h>
|
|
#include <sys/vdev.h>
|
|
#include <sys/txg.h>
|
|
#include <sys/avl.h>
|
|
#include <sys/multilist.h>
|
|
|
|
#ifdef __cplusplus
|
|
extern "C" {
|
|
#endif
|
|
|
|
/*
|
|
* Metaslab allocation tracing record.
|
|
*/
|
|
typedef struct metaslab_alloc_trace {
|
|
list_node_t mat_list_node;
|
|
metaslab_group_t *mat_mg;
|
|
metaslab_t *mat_msp;
|
|
uint64_t mat_size;
|
|
uint64_t mat_weight;
|
|
uint32_t mat_dva_id;
|
|
uint64_t mat_offset;
|
|
int mat_allocator;
|
|
} metaslab_alloc_trace_t;
|
|
|
|
/*
|
|
* Used by the metaslab allocation tracing facility to indicate
|
|
* error conditions. These errors are stored to the offset member
|
|
* of the metaslab_alloc_trace_t record and displayed by mdb.
|
|
*/
|
|
typedef enum trace_alloc_type {
|
|
TRACE_ALLOC_FAILURE = -1ULL,
|
|
TRACE_TOO_SMALL = -2ULL,
|
|
TRACE_FORCE_GANG = -3ULL,
|
|
TRACE_NOT_ALLOCATABLE = -4ULL,
|
|
TRACE_GROUP_FAILURE = -5ULL,
|
|
TRACE_ENOSPC = -6ULL,
|
|
TRACE_CONDENSING = -7ULL,
|
|
TRACE_VDEV_ERROR = -8ULL,
|
|
TRACE_DISABLED = -9ULL,
|
|
} trace_alloc_type_t;
|
|
|
|
#define METASLAB_WEIGHT_PRIMARY (1ULL << 63)
|
|
#define METASLAB_WEIGHT_SECONDARY (1ULL << 62)
|
|
#define METASLAB_WEIGHT_CLAIM (1ULL << 61)
|
|
#define METASLAB_WEIGHT_TYPE (1ULL << 60)
|
|
#define METASLAB_ACTIVE_MASK \
|
|
(METASLAB_WEIGHT_PRIMARY | METASLAB_WEIGHT_SECONDARY | \
|
|
METASLAB_WEIGHT_CLAIM)
|
|
|
|
/*
|
|
* The metaslab weight is used to encode the amount of free space in a
|
|
* metaslab, such that the "best" metaslab appears first when sorting the
|
|
* metaslabs by weight. The weight (and therefore the "best" metaslab) can
|
|
* be determined in two different ways: by computing a weighted sum of all
|
|
* the free space in the metaslab (a space based weight) or by counting only
|
|
* the free segments of the largest size (a segment based weight). We prefer
|
|
* the segment based weight because it reflects how the free space is
|
|
* comprised, but we cannot always use it -- legacy pools do not have the
|
|
* space map histogram information necessary to determine the largest
|
|
* contiguous regions. Pools that have the space map histogram determine
|
|
* the segment weight by looking at each bucket in the histogram and
|
|
* determining the free space whose size in bytes is in the range:
|
|
* [2^i, 2^(i+1))
|
|
* We then encode the largest index, i, that contains regions into the
|
|
* segment-weighted value.
|
|
*
|
|
* Space-based weight:
|
|
*
|
|
* 64 56 48 40 32 24 16 8 0
|
|
* +-------+-------+-------+-------+-------+-------+-------+-------+
|
|
* |PSC1| weighted-free space |
|
|
* +-------+-------+-------+-------+-------+-------+-------+-------+
|
|
*
|
|
* PS - indicates primary and secondary activation
|
|
* C - indicates activation for claimed block zio
|
|
* space - the fragmentation-weighted space
|
|
*
|
|
* Segment-based weight:
|
|
*
|
|
* 64 56 48 40 32 24 16 8 0
|
|
* +-------+-------+-------+-------+-------+-------+-------+-------+
|
|
* |PSC0| idx| count of segments in region |
|
|
* +-------+-------+-------+-------+-------+-------+-------+-------+
|
|
*
|
|
* PS - indicates primary and secondary activation
|
|
* C - indicates activation for claimed block zio
|
|
* idx - index for the highest bucket in the histogram
|
|
* count - number of segments in the specified bucket
|
|
*/
|
|
#define WEIGHT_GET_ACTIVE(weight) BF64_GET((weight), 61, 3)
|
|
#define WEIGHT_SET_ACTIVE(weight, x) BF64_SET((weight), 61, 3, x)
|
|
|
|
#define WEIGHT_IS_SPACEBASED(weight) \
|
|
((weight) == 0 || BF64_GET((weight), 60, 1))
|
|
#define WEIGHT_SET_SPACEBASED(weight) BF64_SET((weight), 60, 1, 1)
|
|
|
|
/*
|
|
* These macros are only applicable to segment-based weighting.
|
|
*/
|
|
#define WEIGHT_GET_INDEX(weight) BF64_GET((weight), 54, 6)
|
|
#define WEIGHT_SET_INDEX(weight, x) BF64_SET((weight), 54, 6, x)
|
|
#define WEIGHT_GET_COUNT(weight) BF64_GET((weight), 0, 54)
|
|
#define WEIGHT_SET_COUNT(weight, x) BF64_SET((weight), 0, 54, x)
|
|
|
|
/*
|
|
* A metaslab class encompasses a category of allocatable top-level vdevs.
|
|
* Each top-level vdev is associated with a metaslab group which defines
|
|
* the allocatable region for that vdev. Examples of these categories include
|
|
* "normal" for data block allocations (i.e. main pool allocations) or "log"
|
|
* for allocations designated for intent log devices (i.e. slog devices).
|
|
* When a block allocation is requested from the SPA it is associated with a
|
|
* metaslab_class_t, and only top-level vdevs (i.e. metaslab groups) belonging
|
|
* to the class can be used to satisfy that request. Allocations are done
|
|
* by traversing the metaslab groups that are linked off of the mc_rotor field.
|
|
* This rotor points to the next metaslab group where allocations will be
|
|
* attempted. Allocating a block is a 3 step process -- select the metaslab
|
|
* group, select the metaslab, and then allocate the block. The metaslab
|
|
* class defines the low-level block allocator that will be used as the
|
|
* final step in allocation. These allocators are pluggable allowing each class
|
|
* to use a block allocator that best suits that class.
|
|
*/
|
|
struct metaslab_class {
|
|
kmutex_t mc_lock;
|
|
spa_t *mc_spa;
|
|
metaslab_group_t *mc_rotor;
|
|
metaslab_ops_t *mc_ops;
|
|
uint64_t mc_aliquot;
|
|
|
|
/*
|
|
* Track the number of metaslab groups that have been initialized
|
|
* and can accept allocations. An initialized metaslab group is
|
|
* one has been completely added to the config (i.e. we have
|
|
* updated the MOS config and the space has been added to the pool).
|
|
*/
|
|
uint64_t mc_groups;
|
|
|
|
/*
|
|
* Toggle to enable/disable the allocation throttle.
|
|
*/
|
|
boolean_t mc_alloc_throttle_enabled;
|
|
|
|
/*
|
|
* The allocation throttle works on a reservation system. Whenever
|
|
* an asynchronous zio wants to perform an allocation it must
|
|
* first reserve the number of blocks that it wants to allocate.
|
|
* If there aren't sufficient slots available for the pending zio
|
|
* then that I/O is throttled until more slots free up. The current
|
|
* number of reserved allocations is maintained by the mc_alloc_slots
|
|
* refcount. The mc_alloc_max_slots value determines the maximum
|
|
* number of allocations that the system allows. Gang blocks are
|
|
* allowed to reserve slots even if we've reached the maximum
|
|
* number of allocations allowed.
|
|
*/
|
|
uint64_t *mc_alloc_max_slots;
|
|
zfs_refcount_t *mc_alloc_slots;
|
|
|
|
uint64_t mc_alloc_groups; /* # of allocatable groups */
|
|
|
|
uint64_t mc_alloc; /* total allocated space */
|
|
uint64_t mc_deferred; /* total deferred frees */
|
|
uint64_t mc_space; /* total space (alloc + free) */
|
|
uint64_t mc_dspace; /* total deflated space */
|
|
uint64_t mc_histogram[RANGE_TREE_HISTOGRAM_SIZE];
|
|
|
|
/*
|
|
* List of all loaded metaslabs in the class, sorted in order of most
|
|
* recent use.
|
|
*/
|
|
multilist_t *mc_metaslab_txg_list;
|
|
};
|
|
|
|
/*
|
|
* Metaslab groups encapsulate all the allocatable regions (i.e. metaslabs)
|
|
* of a top-level vdev. They are linked together to form a circular linked
|
|
* list and can belong to only one metaslab class. Metaslab groups may become
|
|
* ineligible for allocations for a number of reasons such as limited free
|
|
* space, fragmentation, or going offline. When this happens the allocator will
|
|
* simply find the next metaslab group in the linked list and attempt
|
|
* to allocate from that group instead.
|
|
*/
|
|
struct metaslab_group {
|
|
kmutex_t mg_lock;
|
|
metaslab_t **mg_primaries;
|
|
metaslab_t **mg_secondaries;
|
|
avl_tree_t mg_metaslab_tree;
|
|
uint64_t mg_aliquot;
|
|
boolean_t mg_allocatable; /* can we allocate? */
|
|
uint64_t mg_ms_ready;
|
|
|
|
/*
|
|
* A metaslab group is considered to be initialized only after
|
|
* we have updated the MOS config and added the space to the pool.
|
|
* We only allow allocation attempts to a metaslab group if it
|
|
* has been initialized.
|
|
*/
|
|
boolean_t mg_initialized;
|
|
|
|
uint64_t mg_free_capacity; /* percentage free */
|
|
int64_t mg_bias;
|
|
int64_t mg_activation_count;
|
|
metaslab_class_t *mg_class;
|
|
vdev_t *mg_vd;
|
|
taskq_t *mg_taskq;
|
|
metaslab_group_t *mg_prev;
|
|
metaslab_group_t *mg_next;
|
|
|
|
/*
|
|
* In order for the allocation throttle to function properly, we cannot
|
|
* have too many IOs going to each disk by default; the throttle
|
|
* operates by allocating more work to disks that finish quickly, so
|
|
* allocating larger chunks to each disk reduces its effectiveness.
|
|
* However, if the number of IOs going to each allocator is too small,
|
|
* we will not perform proper aggregation at the vdev_queue layer,
|
|
* also resulting in decreased performance. Therefore, we will use a
|
|
* ramp-up strategy.
|
|
*
|
|
* Each allocator in each metaslab group has a current queue depth
|
|
* (mg_alloc_queue_depth[allocator]) and a current max queue depth
|
|
* (mg_cur_max_alloc_queue_depth[allocator]), and each metaslab group
|
|
* has an absolute max queue depth (mg_max_alloc_queue_depth). We
|
|
* add IOs to an allocator until the mg_alloc_queue_depth for that
|
|
* allocator hits the cur_max. Every time an IO completes for a given
|
|
* allocator on a given metaslab group, we increment its cur_max until
|
|
* it reaches mg_max_alloc_queue_depth. The cur_max resets every txg to
|
|
* help protect against disks that decrease in performance over time.
|
|
*
|
|
* It's possible for an allocator to handle more allocations than
|
|
* its max. This can occur when gang blocks are required or when other
|
|
* groups are unable to handle their share of allocations.
|
|
*/
|
|
uint64_t mg_max_alloc_queue_depth;
|
|
uint64_t *mg_cur_max_alloc_queue_depth;
|
|
zfs_refcount_t *mg_alloc_queue_depth;
|
|
int mg_allocators;
|
|
/*
|
|
* A metalab group that can no longer allocate the minimum block
|
|
* size will set mg_no_free_space. Once a metaslab group is out
|
|
* of space then its share of work must be distributed to other
|
|
* groups.
|
|
*/
|
|
boolean_t mg_no_free_space;
|
|
|
|
uint64_t mg_allocations;
|
|
uint64_t mg_failed_allocations;
|
|
uint64_t mg_fragmentation;
|
|
uint64_t mg_histogram[RANGE_TREE_HISTOGRAM_SIZE];
|
|
|
|
int mg_ms_disabled;
|
|
boolean_t mg_disabled_updating;
|
|
kmutex_t mg_ms_disabled_lock;
|
|
kcondvar_t mg_ms_disabled_cv;
|
|
};
|
|
|
|
/*
|
|
* This value defines the number of elements in the ms_lbas array. The value
|
|
* of 64 was chosen as it covers all power of 2 buckets up to UINT64_MAX.
|
|
* This is the equivalent of highbit(UINT64_MAX).
|
|
*/
|
|
#define MAX_LBAS 64
|
|
|
|
/*
|
|
* Each metaslab maintains a set of in-core trees to track metaslab
|
|
* operations. The in-core free tree (ms_allocatable) contains the list of
|
|
* free segments which are eligible for allocation. As blocks are
|
|
* allocated, the allocated segment are removed from the ms_allocatable and
|
|
* added to a per txg allocation tree (ms_allocating). As blocks are
|
|
* freed, they are added to the free tree (ms_freeing). These trees
|
|
* allow us to process all allocations and frees in syncing context
|
|
* where it is safe to update the on-disk space maps. An additional set
|
|
* of in-core trees is maintained to track deferred frees
|
|
* (ms_defer). Once a block is freed it will move from the
|
|
* ms_freed to the ms_defer tree. A deferred free means that a block
|
|
* has been freed but cannot be used by the pool until TXG_DEFER_SIZE
|
|
* transactions groups later. For example, a block that is freed in txg
|
|
* 50 will not be available for reallocation until txg 52 (50 +
|
|
* TXG_DEFER_SIZE). This provides a safety net for uberblock rollback.
|
|
* A pool could be safely rolled back TXG_DEFERS_SIZE transactions
|
|
* groups and ensure that no block has been reallocated.
|
|
*
|
|
* The simplified transition diagram looks like this:
|
|
*
|
|
*
|
|
* ALLOCATE
|
|
* |
|
|
* V
|
|
* free segment (ms_allocatable) -> ms_allocating[4] -> (write to space map)
|
|
* ^
|
|
* | ms_freeing <--- FREE
|
|
* | |
|
|
* | v
|
|
* | ms_freed
|
|
* | |
|
|
* +-------- ms_defer[2] <-------+-------> (write to space map)
|
|
*
|
|
*
|
|
* Each metaslab's space is tracked in a single space map in the MOS,
|
|
* which is only updated in syncing context. Each time we sync a txg,
|
|
* we append the allocs and frees from that txg to the space map. The
|
|
* pool space is only updated once all metaslabs have finished syncing.
|
|
*
|
|
* To load the in-core free tree we read the space map from disk. This
|
|
* object contains a series of alloc and free records that are combined
|
|
* to make up the list of all free segments in this metaslab. These
|
|
* segments are represented in-core by the ms_allocatable and are stored
|
|
* in an AVL tree.
|
|
*
|
|
* As the space map grows (as a result of the appends) it will
|
|
* eventually become space-inefficient. When the metaslab's in-core
|
|
* free tree is zfs_condense_pct/100 times the size of the minimal
|
|
* on-disk representation, we rewrite it in its minimized form. If a
|
|
* metaslab needs to condense then we must set the ms_condensing flag to
|
|
* ensure that allocations are not performed on the metaslab that is
|
|
* being written.
|
|
*/
|
|
struct metaslab {
|
|
/*
|
|
* This is the main lock of the metaslab and its purpose is to
|
|
* coordinate our allocations and frees [e.g metaslab_block_alloc(),
|
|
* metaslab_free_concrete(), ..etc] with our various syncing
|
|
* procedures [e.g. metaslab_sync(), metaslab_sync_done(), ..etc].
|
|
*
|
|
* The lock is also used during some miscellaneous operations like
|
|
* using the metaslab's histogram for the metaslab group's histogram
|
|
* aggregation, or marking the metaslab for initialization.
|
|
*/
|
|
kmutex_t ms_lock;
|
|
|
|
/*
|
|
* Acquired together with the ms_lock whenever we expect to
|
|
* write to metaslab data on-disk (i.e flushing entries to
|
|
* the metaslab's space map). It helps coordinate readers of
|
|
* the metaslab's space map [see spa_vdev_remove_thread()]
|
|
* with writers [see metaslab_sync() or metaslab_flush()].
|
|
*
|
|
* Note that metaslab_load(), even though a reader, uses
|
|
* a completely different mechanism to deal with the reading
|
|
* of the metaslab's space map based on ms_synced_length. That
|
|
* said, the function still uses the ms_sync_lock after it
|
|
* has read the ms_sm [see relevant comment in metaslab_load()
|
|
* as to why].
|
|
*/
|
|
kmutex_t ms_sync_lock;
|
|
|
|
kcondvar_t ms_load_cv;
|
|
space_map_t *ms_sm;
|
|
uint64_t ms_id;
|
|
uint64_t ms_start;
|
|
uint64_t ms_size;
|
|
uint64_t ms_fragmentation;
|
|
|
|
range_tree_t *ms_allocating[TXG_SIZE];
|
|
range_tree_t *ms_allocatable;
|
|
uint64_t ms_allocated_this_txg;
|
|
uint64_t ms_allocating_total;
|
|
|
|
/*
|
|
* The following range trees are accessed only from syncing context.
|
|
* ms_free*tree only have entries while syncing, and are empty
|
|
* between syncs.
|
|
*/
|
|
range_tree_t *ms_freeing; /* to free this syncing txg */
|
|
range_tree_t *ms_freed; /* already freed this syncing txg */
|
|
range_tree_t *ms_defer[TXG_DEFER_SIZE];
|
|
range_tree_t *ms_checkpointing; /* to add to the checkpoint */
|
|
|
|
/*
|
|
* The ms_trim tree is the set of allocatable segments which are
|
|
* eligible for trimming. (When the metaslab is loaded, it's a
|
|
* subset of ms_allocatable.) It's kept in-core as long as the
|
|
* autotrim property is set and is not vacated when the metaslab
|
|
* is unloaded. Its purpose is to aggregate freed ranges to
|
|
* facilitate efficient trimming.
|
|
*/
|
|
range_tree_t *ms_trim;
|
|
|
|
boolean_t ms_condensing; /* condensing? */
|
|
boolean_t ms_condense_wanted;
|
|
|
|
/*
|
|
* The number of consumers which have disabled the metaslab.
|
|
*/
|
|
uint64_t ms_disabled;
|
|
|
|
/*
|
|
* We must always hold the ms_lock when modifying ms_loaded
|
|
* and ms_loading.
|
|
*/
|
|
boolean_t ms_loaded;
|
|
boolean_t ms_loading;
|
|
kcondvar_t ms_flush_cv;
|
|
boolean_t ms_flushing;
|
|
|
|
/*
|
|
* The following histograms count entries that are in the
|
|
* metaslab's space map (and its histogram) but are not in
|
|
* ms_allocatable yet, because they are in ms_freed, ms_freeing,
|
|
* or ms_defer[].
|
|
*
|
|
* When the metaslab is not loaded, its ms_weight needs to
|
|
* reflect what is allocatable (i.e. what will be part of
|
|
* ms_allocatable if it is loaded). The weight is computed from
|
|
* the spacemap histogram, but that includes ranges that are
|
|
* not yet allocatable (because they are in ms_freed,
|
|
* ms_freeing, or ms_defer[]). Therefore, when calculating the
|
|
* weight, we need to remove those ranges.
|
|
*
|
|
* The ranges in the ms_freed and ms_defer[] range trees are all
|
|
* present in the spacemap. However, the spacemap may have
|
|
* multiple entries to represent a contiguous range, because it
|
|
* is written across multiple sync passes, but the changes of
|
|
* all sync passes are consolidated into the range trees.
|
|
* Adjacent ranges that are freed in different sync passes of
|
|
* one txg will be represented separately (as 2 or more entries)
|
|
* in the space map (and its histogram), but these adjacent
|
|
* ranges will be consolidated (represented as one entry) in the
|
|
* ms_freed/ms_defer[] range trees (and their histograms).
|
|
*
|
|
* When calculating the weight, we can not simply subtract the
|
|
* range trees' histograms from the spacemap's histogram,
|
|
* because the range trees' histograms may have entries in
|
|
* higher buckets than the spacemap, due to consolidation.
|
|
* Instead we must subtract the exact entries that were added to
|
|
* the spacemap's histogram. ms_synchist and ms_deferhist[]
|
|
* represent these exact entries, so we can subtract them from
|
|
* the spacemap's histogram when calculating ms_weight.
|
|
*
|
|
* ms_synchist represents the same ranges as ms_freeing +
|
|
* ms_freed, but without consolidation across sync passes.
|
|
*
|
|
* ms_deferhist[i] represents the same ranges as ms_defer[i],
|
|
* but without consolidation across sync passes.
|
|
*/
|
|
uint64_t ms_synchist[SPACE_MAP_HISTOGRAM_SIZE];
|
|
uint64_t ms_deferhist[TXG_DEFER_SIZE][SPACE_MAP_HISTOGRAM_SIZE];
|
|
|
|
/*
|
|
* Tracks the exact amount of allocated space of this metaslab
|
|
* (and specifically the metaslab's space map) up to the most
|
|
* recently completed sync pass [see usage in metaslab_sync()].
|
|
*/
|
|
uint64_t ms_allocated_space;
|
|
int64_t ms_deferspace; /* sum of ms_defermap[] space */
|
|
uint64_t ms_weight; /* weight vs. others in group */
|
|
uint64_t ms_activation_weight; /* activation weight */
|
|
|
|
/*
|
|
* Track of whenever a metaslab is selected for loading or allocation.
|
|
* We use this value to determine how long the metaslab should
|
|
* stay cached.
|
|
*/
|
|
uint64_t ms_selected_txg;
|
|
/*
|
|
* ms_load/unload_time can be used for performance monitoring
|
|
* (e.g. by dtrace or mdb).
|
|
*/
|
|
hrtime_t ms_load_time; /* time last loaded */
|
|
hrtime_t ms_unload_time; /* time last unloaded */
|
|
hrtime_t ms_selected_time; /* time last allocated from */
|
|
|
|
uint64_t ms_alloc_txg; /* last successful alloc (debug only) */
|
|
uint64_t ms_max_size; /* maximum allocatable size */
|
|
|
|
/*
|
|
* -1 if it's not active in an allocator, otherwise set to the allocator
|
|
* this metaslab is active for.
|
|
*/
|
|
int ms_allocator;
|
|
boolean_t ms_primary; /* Only valid if ms_allocator is not -1 */
|
|
|
|
/*
|
|
* The metaslab block allocators can optionally use a size-ordered
|
|
* range tree and/or an array of LBAs. Not all allocators use
|
|
* this functionality. The ms_allocatable_by_size should always
|
|
* contain the same number of segments as the ms_allocatable. The
|
|
* only difference is that the ms_allocatable_by_size is ordered by
|
|
* segment sizes.
|
|
*/
|
|
zfs_btree_t ms_allocatable_by_size;
|
|
zfs_btree_t ms_unflushed_frees_by_size;
|
|
uint64_t ms_lbas[MAX_LBAS];
|
|
|
|
metaslab_group_t *ms_group; /* metaslab group */
|
|
avl_node_t ms_group_node; /* node in metaslab group tree */
|
|
txg_node_t ms_txg_node; /* per-txg dirty metaslab links */
|
|
avl_node_t ms_spa_txg_node; /* node in spa_metaslabs_by_txg */
|
|
/*
|
|
* Node in metaslab class's selected txg list
|
|
*/
|
|
multilist_node_t ms_class_txg_node;
|
|
|
|
/*
|
|
* Allocs and frees that are committed to the vdev log spacemap but
|
|
* not yet to this metaslab's spacemap.
|
|
*/
|
|
range_tree_t *ms_unflushed_allocs;
|
|
range_tree_t *ms_unflushed_frees;
|
|
|
|
/*
|
|
* We have flushed entries up to but not including this TXG. In
|
|
* other words, all changes from this TXG and onward should not
|
|
* be in this metaslab's space map and must be read from the
|
|
* log space maps.
|
|
*/
|
|
uint64_t ms_unflushed_txg;
|
|
|
|
/* updated every time we are done syncing the metaslab's space map */
|
|
uint64_t ms_synced_length;
|
|
|
|
boolean_t ms_new;
|
|
};
|
|
|
|
typedef struct metaslab_unflushed_phys {
|
|
/* on-disk counterpart of ms_unflushed_txg */
|
|
uint64_t msp_unflushed_txg;
|
|
} metaslab_unflushed_phys_t;
|
|
|
|
#ifdef __cplusplus
|
|
}
|
|
#endif
|
|
|
|
#endif /* _SYS_METASLAB_IMPL_H */
|